|
Home
Research
Teaching
Broader
Educational Activities
List
of Publications
Graduate Students and Visiting Scholars
|
Research
Interests
There are
two general research areas that have remained separate but ideas and
methodologies developed in one have crossed over to enrich each other.
Granular Materials
Granular materials are seen in our daily lives such as
cereals and grains, pharmaceutical products in powders and pills, industrial
raw or processed materials from coal to a wide range of minerals, and
the all over soil. Granular materials are not like air, water, or rocks
with a well-defined material response to load. It can change its behavior
dramatically by a slight change of the solid fraction. The collapse
of a dam and the jamming of silos are examples.
Project 1: Consolidation
of insulation materials
Cryogenic liquid storage tanks are constructed by a double-layer sphere.
The interior storage contains the liquid. To insulate it from
the outside temperature, the hollow space between the interior
storage tank and the exterior container is filled with a powder
material. Over the lifetime of the storage, periodic thermal expansion/contraction
of the interior tank compacts the powder in the hollow space downwards, exposing
the top and reducing the insulation effectiveness. We simulate
this process by modeling the physical properties of the powder
particles to evaluate the rate of compaction. (Click the picture
to see an animation.)
|
|
|
Project 2: Modeling irregular
shape particles
Design of construction equipment is challenging because the materials
it has to handle is not as simple as a pure liquid. This is one
of the major reasons for industries that deal with particulate
materials to under-perform than their counterparts who deal with
liquid materials. Lacking a reliable constitutive law to describe
these granular materials, computer simulations with good descriptions
of the particle’s physical properties can help enormously. We
use overlapping spheres to study the effect particle shape on
the bulk friction behavior by comparing with results from direct
shear box physical tests. (Click the picture to see an animation.)
|
|
|
Project 3: Lunar soil processing
Construction on earth has a very long history prior to any scientific
knowledge of the soil behavior. Its success has come from experience
of many lessons learned from failures. To explore other celestial
bodies and planets for resources, ingenious engineering based
on scientific knowledge is needed. In this project we study the
transport of granular materials such as a soil in hoppers and
pipes. They represent the most basic industrial processing equipment
for granular materials. Electrostatic forces between particles
are considered under either earth or moon gravity. Can you guess
which is which in the two figures on the right? (Click
for the answer.)
|
|
|
Project 4: Rheological
properties of granular materials
Everyone knows that the viscosity of a liquid is a well-defined property.
It can be measured by several standard methods with off-the-shelve
instruments. The most common one is a Couette-device.
This device is a concentric cylinder with a narrow gap. The liquid
to be measured fills this gap. The device has one fixed wall and
one moving wall. A fixed speed is applied to move one wall and
measure the required torque. The relation between the torque and
the speed of the motion are then used to measure the viscosity.
For granular materials, similar device has been constructed. It
was found that the “viscosity” obtained was not only dependent
on the materials, but also on the rate of shear and the solid
fraction. In this study we investigate the effect of many micromechanical
level parameters on the bulk behavior: particle size, shape, boundary
condition, and the contact properties between particles. (Click
the picture to see an animation.)
|
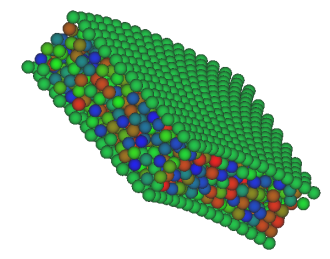 |
Sea
Ice Dynamics
Adding both polar regions together, in average sea ice
covers about 20 million square kilometers, roughly 5% of the ocean surface.
http://en.wikipedia.org/wiki/Polar_ice_packs
Although this does not seem a large fraction, sea ice drives
the world climate directly. The Antarctic sea ice formation is associated
with large amount of salt ejection. The heavy salty water sinks like
a plume that drives a lateral ocean current. This
current moves northward into the Pacific Ocean, circles around,
back through the Southern Ocean, up to the Atlantic Ocean and down again.
Without this current, northern Europe would be much colder then
it is now. Sea ice also responds to the climate change, as we observe
the decline of summer ice cover in the Arctic. As the Arctic ice cover
shrinks, there are many opportunities for economic development in the
region. These opportunities also bring concerns of their environmental
impact. Sea ice dynamics is therefore subject to serious research investigations.
Project 1: Sea ice rheology
Sea ice is not a uniform homogeneous material. Its physical and mechanical
properties depend on its salinity and temperature at the crystal
scale and on its thickness and thickness distribution, floe size
and size distribution, and concentration, at the geophysical scale.
To be able to predict the motion and deformation of a sea ice
cover, one must know how to relate the deformation to forcing.
As shown in the pictures on the right, sea ice cover can range
from a slush to an aggregate to various
floes. These floe can be fairly large and concentrated until they
simply pack together to form a consolidated sheet with extremely
heterogeneous thickness distribution. Each of such sea ice cover
has a different mechanical property. This study focused on the
sea ice covers represented by a dispersed aggregate such as shown
in pictures 2 and 3. Recognizing such composition of sea ice is
simply a two dimensional granular material, one may using the
granular materials approach to derive the stress and strain-rate
relationship. The resulting rheology is more appropriate for the
marginal ice zone with fragmented ice floes dispersed in open
water. (http://onlinelibrary.wiley.com/doi/10.1029/JC092iC07p07085/abstract)
|
|
|
Project 2: Sea ice drift in a wave field
Motion of ice floes in the ocean is known to be driven by wind and current.
The additional drift due to waves has been investigated in this
project. The mechanism of how waves move a floating piece of ice
is the same as the sliding of an object on a slope, provided that
the ice floe is much smaller than the wavelength. For very large
floes such as an ice berg, the mechanism is different. There the
reflection of the wave momentum creates an equivalent force. The
slope-sliding mechanism is compounded with drag and added inertia
effects of the accelerating ice floe to determine it motion. While
still oscillatory due to the wave motion, there is net forward
drift of the ice floes. Being much larger than water molecules,
this drift is different from the well-known Stokes drift, and
is dependent on the floe size and its drag and inertia coefficients.
(http://cedb.asce.org/cgi/WWWdisplay.cgi?129268).
Click here to see an animation
|
|
|
Project 3: Ice motion measured from AVHRR imagery
Remote sensing is a crucial part of sea ice study. It provides continuous
observations over a large area of the earth surface. There are
many different sensors of various capabilities onboard many satellites.
In this study we use the Advanced Very High Resolution Radiometer
(AVHRR) imageries to track the motion of ice features in the Sea
of Okhotsk. AVHRR can provide images for cloud-free regions because
it can sense from red to far-infrared bands. We use two consecutive
images 12-hr apart of the same region. By an automated feature
identification computer algorithm we can map out the displacement
vectors over the entire ice field. This method was applied to
the Sea of Okhotsk for several winters. A sample picture of the
displacement field over the 12-hr span is shown on the right.
Connecting these displacement fields over time, one can track
the origin of ice at a location of interest. Statistical analysis
of such information provides site evaluation of offshore structures
and coastal impacts. (Click here to see a related publication.)
|
|
|
Project 4: Sea ice formation in a wave field
Cooling below the freezing temperature turns water to ice. In calm water
such as over small lakes without the disturbance of wind, ice
forms as a smooth sheet first, then crystal
grows under the surface ice to form vertical structures called
columnar ice. If wave is present, as in large lakes and the ocean,
a different process takes over. Ice first forms into a
slush with random crystal orientations. The slush congeals
into pancake shape ice floes, which grow in time by incorporating
the slush surrounding them and by freezing together with each
other. The size of these pancake floes are strikingly uniform,
as shown in the picture to the right (courtesy of Tony Worby,
Australian Antarctic
Division). In this project we investigate what controls
this uniform size. Furthermore, under stormy conditions waves
may raft the existing pancake ice floes together to mechanically
thicken the ice cover. The thickening process is much faster than
the thermal growth. However, thickening cannot proceed indefinitely
because the resistance of the stacked up ice floes progressively
makes the rafting more difficult. The equilibrium thickness of
an ice cover under this rafting process is also investigated via
laboratory and computer simulated experiments. (Click each picture
to see an associate animation.)
|
|
|
Project 5: Sea ice and wave interaction
As wave propagates under an ice cover, it changes its velocity: both
the speed and direction. This phenomenon is called refraction
and is well-known in optics. In gravity waves, this phenomenon
is related to the mechanical properties of the ice cover. On a
geophysical scale, the mechanical properties of various ice covers
are not characterized. Existing theories for gravity wave propagation
under an ice cover either model ice cover as an
elastic or a viscous material. In this study, a viscoelastic
model is investigated. The purpose is to set up a simplest approach
to unify the range of different ice types as shown in Project
1, where evidently picture 1 is like a viscous material and picture
4 is like a (imperfect) elastic material. The two in between very
likely possess both material characteristics. In this project
we determine not only the refraction property of gravity waves
under different ice covers parameterized by a range of viscosity
and elasticity, but also the attenuation of the wave energy, the
reflection and transmission between ice covers of different viscoelastic
properties. (Click here to see related publications.)
|
|
|
Project 6: Offshore structure under ice and wave actions
In this project we study the additional load, force and torque, due
to impact of pancake ice on cylinders. Many offshore structures
have successfully operated in open water conditions subject to
wind, wave, and currents. In ice-infested regions, wave is reduced
due to the attenuation effect from the ice cover,
however, the motion of ice itself becomes a new concern. Since
this wave induced ice actions are oscillatory, they may invoke
resonance of the offshore structure. Even if the vibration does
not pose threat to the structures, it is uncomfortable for workers.
We apply a computer simulation as in Project 4 to study the impact
frequency spectrum under different ice conditions. (Click here to see a related
publication.) (Click the picture to see an animation.)
|
|
Project 7: Laboratory and field study of sea ice
Field study of sea ice is expensive because it requires large ships
to travel to a remote location. Laboratory study is a valuable
approach to obtain data under a well-controlled environment. We
have used the Army Cold Regions Research and Engineering Laboratory
(CRREL)
and the Hamburg Ship Research Basin (HSVA)
facilities from 1995 to 2008 to conduct a series of experiments.
These experiments enabled us to identify the formation of pancake
ice, the controlling factors of its growth dynamics, the rate
of formation, and the wave propagation under pancake ice covers.
On the right are two photos of the laboratory facilities at CRREL
(the black and white one, click the photo to see a video) and
at HSVA (the color one, click the photo to see a video). Photo
of a graduate student (Maggie) in the sea next to an iceberg,
and our research team (Dr. Toyota, two graduate students: Yong
and Mingrui, and Hayley Shen) at HSVA.
|
|
|
|